Basic Neurophysiology: Difference between revisions
(9 intermediate revisions by one other user not shown) | |||
Line 13: | Line 13: | ||
An action potential is generated near the axon hillock when the threshold is reached (-65 mV), the signal is then propagated down (travels) the axon. Active axonal conduction is due to the diffusion of Na+ into the cell which creates waves of depolarization whereas passive axonal conduction occurs as the signal travels down a myelinated axon along each segment, an action potential is generated at each node of Ranvier between the segments (Pinel, 2017) propagated from one node to the next (saltatory conduction). | An action potential is generated near the axon hillock when the threshold is reached (-65 mV), the signal is then propagated down (travels) the axon. Active axonal conduction is due to the diffusion of Na+ into the cell which creates waves of depolarization whereas passive axonal conduction occurs as the signal travels down a myelinated axon along each segment, an action potential is generated at each node of Ranvier between the segments (Pinel, 2017) propagated from one node to the next (saltatory conduction). | ||
[[File:ion-channels2.png|center|974px|560px]] | |||
(Source: Wikipedia, https://en.wikipedia.org/wiki/Saltatory_conduction) | |||
Axons that are wrapped in myelin (fatty substance), which serves as insulation of the signal, have faster communication than unmyelinated axons. The nodes of Ranvier are unmyelinated areas on the axon where the ions flow (e.g., Na+, K+). | Axons that are wrapped in myelin (fatty substance), which serves as insulation of the signal, have faster communication than unmyelinated axons. The nodes of Ranvier are unmyelinated areas on the axon where the ions flow (e.g., Na+, K+). | ||
Line 19: | Line 23: | ||
The process by which neurons communicate with each other is synaptic transmission which occurs across synapses using either electrical or chemical signals. Electrical signaling occurs as an action potential travels from the pre- to the postsynaptic neuron via gap junctions. However, most synaptic transmission involves chemical signals. These chemicals are neurotransmitters, molecules which can function either directly (ionotropic) or indirectly via second messengers (metabotropic). The steps in chemical neurotransmission are: synthesis of the neurotransmitter (in the soma), storage of the neurotransmitter in the vesicles, release into the synapse, binding to the receptor on the postsynaptic neuron, and inactivation of the neurotransmitter via reuptake or enzymatic degradation which terminates the action of the neurotransmitter in the synapse. | The process by which neurons communicate with each other is synaptic transmission which occurs across synapses using either electrical or chemical signals. Electrical signaling occurs as an action potential travels from the pre- to the postsynaptic neuron via gap junctions. However, most synaptic transmission involves chemical signals. These chemicals are neurotransmitters, molecules which can function either directly (ionotropic) or indirectly via second messengers (metabotropic). The steps in chemical neurotransmission are: synthesis of the neurotransmitter (in the soma), storage of the neurotransmitter in the vesicles, release into the synapse, binding to the receptor on the postsynaptic neuron, and inactivation of the neurotransmitter via reuptake or enzymatic degradation which terminates the action of the neurotransmitter in the synapse. | ||
[[File:synapses.png|center|600px|387px]] | |||
(Source: http://biology4alevel.blogspot.com/2016/06/122-synapses.html) | |||
== Recording Techniques == | == Recording Techniques == | ||
Line 35: | Line 43: | ||
=== Signal to Noise Ratio === | === Signal to Noise Ratio === | ||
Signal to noise ratio refers to the electrical or | Signal to noise ratio refers to the electrical or neurophysiological signal and the concurrent interference (noise). Interference occurs both naturally and artificially. For example, “noise” from the body (extraneous biological activity) or perisurgical such as the use of instrumentation or electrical interference. The amplitude of the signal and the amplitude of the noise overlap given that the frequency content of noise contains a myriad of all frequencies and the specific frequency content of the signal would be within that range of all frequencies (Zouridakis and Papanicoloau, 2000). Therefore, to increase specificity and detection of the electrical signal, the signal to noise ratio, the interference must be dampened which can be accomplished using filters as described above and the signal may be increased using averaging. | ||
=== Signal Averaging === | === Signal Averaging === | ||
Line 49: | Line 57: | ||
==References== | ==References== | ||
Hodgkin AL and Huxley AF. "A quantitative description of membrane current and its application to conduction and excitation in nerve". The Journal of Physiology 117.4 (1952): 500–544. | # Hodgkin AL and Huxley AF. "A quantitative description of membrane current and its application to conduction and excitation in nerve". The Journal of Physiology 117.4 (1952): 500–544. | ||
# Moller, A. (1995). Intraoperative Neurophysiologic Monitoring. Informa Healthcare. | |||
Moller, A. (1995). Intraoperative Neurophysiologic Monitoring. Informa Healthcare. | # Pinel, J. P. J. (2014). Biopsychology. Harlow, Essex: Pearson Education Limited. | ||
# Zouridakis, G. and Papanicoloau, A.C. (2000). A Concise Guide to Intraoperative Monitoring. CRC Press: Taylor and Francis Group. | |||
Pinel, J. P. J. (2014). Biopsychology. Harlow, Essex: Pearson Education Limited. | |||
Zouridakis, G. and Papanicoloau, A.C. (2000). A Concise Guide to Intraoperative Monitoring. CRC Press: Taylor and Francis Group. |
Latest revision as of 18:59, 18 August 2021
Neurophysiology is the study of the function of the nervous system.
Membrane Ion Channels
All animal cells are enclosed in a plasma membrane, which separates its cytoplasm with the extracellular environment. Cell membrane has the structure of a lipid bilayer, with large molecules embedded in it. Concentration of ions are different across the cell membrane, with more sodium ions (Na+) and chloride ions (Cl-) outside the cell, and more potassium ions (K+) and negative charged protein molecules (A-) inside the cell. Selective ion channels at rest allow potassium ions (K+) cross the membrane easily, creating a voltage more negative inside the cell than outside, which is called resting membrane potential. The resting membrane potential of a neuron is about -70 mV.
Action Potential
An action potential is the change in electrical potential associated with the passage of an impulse along the membrane of a muscle cell or nerve cell in response to a stimulus, which occurs as the electrical potential briefly (about 1ms) rises and falls (Hodgkin and Huxley). Specifically, an action potential occurs when the threshold is reached (-65 mV) which activates the voltage-gated ion channels to open. When the threshold is reached: Na+ channels open and Na+ rushes into the cell while K+ channels open slowly and K+ leaves the cell, the cell then becomes hyperpolarized (Pinel, 2017). There are three phases of an action potential: the rising phase (Na+ and K+ channels open), repolarization (Na+ channels close), and hyperpolarization (K+ channels start to close).
Propagated Neural Activity
An action potential is generated near the axon hillock when the threshold is reached (-65 mV), the signal is then propagated down (travels) the axon. Active axonal conduction is due to the diffusion of Na+ into the cell which creates waves of depolarization whereas passive axonal conduction occurs as the signal travels down a myelinated axon along each segment, an action potential is generated at each node of Ranvier between the segments (Pinel, 2017) propagated from one node to the next (saltatory conduction).
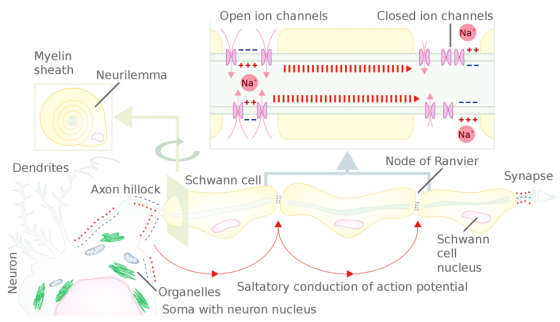
(Source: Wikipedia, https://en.wikipedia.org/wiki/Saltatory_conduction)
Axons that are wrapped in myelin (fatty substance), which serves as insulation of the signal, have faster communication than unmyelinated axons. The nodes of Ranvier are unmyelinated areas on the axon where the ions flow (e.g., Na+, K+).
Synaptic Transmission
The process by which neurons communicate with each other is synaptic transmission which occurs across synapses using either electrical or chemical signals. Electrical signaling occurs as an action potential travels from the pre- to the postsynaptic neuron via gap junctions. However, most synaptic transmission involves chemical signals. These chemicals are neurotransmitters, molecules which can function either directly (ionotropic) or indirectly via second messengers (metabotropic). The steps in chemical neurotransmission are: synthesis of the neurotransmitter (in the soma), storage of the neurotransmitter in the vesicles, release into the synapse, binding to the receptor on the postsynaptic neuron, and inactivation of the neurotransmitter via reuptake or enzymatic degradation which terminates the action of the neurotransmitter in the synapse.
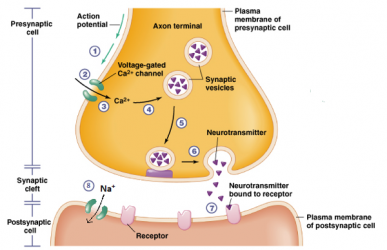
(Source: http://biology4alevel.blogspot.com/2016/06/122-synapses.html)
Recording Techniques
Electrodes
Electrodes are used to deliver and record electrical stimuli. In Neurophysiology, electrodes deliver auditory, visual, and electrical stimuli, the amplitude, duration, and rate of which can be varied. Various types of electrodes are used for recording electrophysiological activity from the scalp, nerves, and muscles (Zouridakis and Papanicoloau, 2000). There are various types of electrodes such as surface electrodes with hydrogel pads, subdermal needle electrodes, and EEG cups.
Amplifiers
An amplifier multiplies the input voltage to result in a greater output voltage (amplifier is the circuit and the difference between two input voltages). Specifically, the amplifier functions as the power and converts alternating current to direct current, thereby flowing in only one direction, a resistor then increases the current flow by reducing the resistance. The signal dictates the amount of the current, such that a greater signal allows for more current and more current results in more amplitude of the signal.
Filtering
Filters are used to determine the bandwidth while defining the specific content of the frequencies from the recorded signals, thereby attenuating some frequencies in the signal. Filters can manipulate latency and amplitude. There are different types of filters including high pass (attenuate high frequencies) and low pass filters (attenuate low frequencies). High frequency filters will remove all frequencies above a set value (e.g., 5Hz), similarly, low frequency filters will remove frequencies below a set value (e.g., 5Hz). Another type of filter is the notch filter which will remove one frequency such as the 60Hz, the background or external frequency that can interfere with the recorded neurophysiological signals as it is the frequency of the alternating current used in the power grid.
Signal to Noise Ratio
Signal to noise ratio refers to the electrical or neurophysiological signal and the concurrent interference (noise). Interference occurs both naturally and artificially. For example, “noise” from the body (extraneous biological activity) or perisurgical such as the use of instrumentation or electrical interference. The amplitude of the signal and the amplitude of the noise overlap given that the frequency content of noise contains a myriad of all frequencies and the specific frequency content of the signal would be within that range of all frequencies (Zouridakis and Papanicoloau, 2000). Therefore, to increase specificity and detection of the electrical signal, the signal to noise ratio, the interference must be dampened which can be accomplished using filters as described above and the signal may be increased using averaging.
Signal Averaging
Evoked potentials elicited by electrical signals are small and a summation and average are thus necessary for detection of the signal (Zouridakis and Papanicoloau, 2000). Specifically, the process of averaging is used to record evoked potentials, the electrical response in the brain to repeated sensory stimuli. Given that the individual electrical responses in response to stimuli are small and therefore difficult to distinguish, and that background signals (e.g., ongoing and simultaneous brain activity, other electrical signals) can interfere with this detection, it is assumed that the signal of interest is embedded within all other ongoing electrical activity (noise), therefore summing and averaging improves (increases) the signal to noise ratio by reducing the noise amplitude when the signal to noise ratio (expressed in decibels) is low; the higher the number of trials that are averaged, the lower the noise.
The time (ms) just before and after the presentation of the stimulus is known as an epoch. The averaging procedure involves repeated stimulation and signal recording such that each preceding response is added to the most recent (and at each time point), and those values are then divided. That is, the epoch of each time point is added to all preceding ones and that sum is divided by the total number of epochs (trials). For multiple channels recording simultaneously, each channel is averaged independently resulting in a separate evoked potential for each channel. Evoked responses (e.g., sensory or motor) are averaged responses whereas electrically triggered EMG are nonaveraged.
Electrical Safety
There are many sources of electrical activity in the operating theater. The patient must be protected from the external sources of electrical activity. One way the patient is protected is by the use of the ground wire (placed subcutaneously) in addition to the needle electrodes that are delivering stimulation. Also, the neuromonitoring equipment is able to be quickly accessed and detached from the patient, specifically the electrodes can be removed from the cables and/or the cables can be detached from the computer (Moller, 1995).
References
- Hodgkin AL and Huxley AF. "A quantitative description of membrane current and its application to conduction and excitation in nerve". The Journal of Physiology 117.4 (1952): 500–544.
- Moller, A. (1995). Intraoperative Neurophysiologic Monitoring. Informa Healthcare.
- Pinel, J. P. J. (2014). Biopsychology. Harlow, Essex: Pearson Education Limited.
- Zouridakis, G. and Papanicoloau, A.C. (2000). A Concise Guide to Intraoperative Monitoring. CRC Press: Taylor and Francis Group.